ABSTRACT
While the non-linearity of the Stefan-Boltzmann equation leads to at least a 60 deg. C overestimate of the Moon’s average surface temperature if a global-average solar flux is used in place of computing temperatures over a sphere with a diurnal cycle, the error is only about 5 deg. C for the Earth. The difference is due the the very long lunar day (29.5 Earth days), which causes a very large diurnal cycle in temperature, which enhances the errors arising from the nonlinearity of the S-B equation.
The greenhouse effect is often claimed to cause an average warming of the Earth’s surface of about 33 deg. C, from an atmosphere-free value of about 255 K to the observed value of around 288 K. In the no-atmosphere case, the absorbed solar flux heats the surface up until the thermal emission of longwave radiation matches the intensity of absorbed sunlight.
Typically this theoretical average surface temperature is computed using a global average of the absorbed solar flux, and then using the Stefan-Boltzmann equation to find the emitting temperature that matches it.
But the strong nonlinearity of how the S-B flux depends upon temperature can lead to a warm bias in the no-atmosphere temperature estimate if a wide range of solar fluxes are used in a single average:
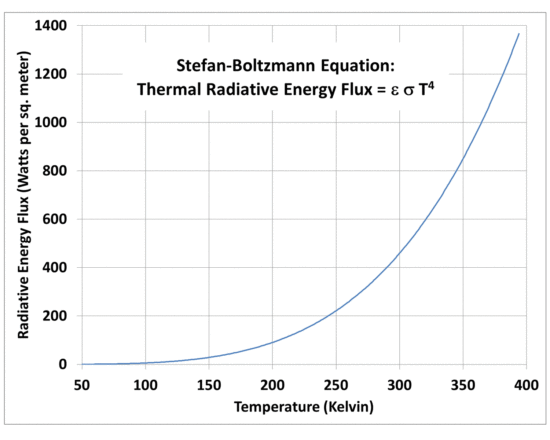
Fig. 1. The non-linearity of the Stefan-Boltzmann equation leads to a warm bias if a global average solar flux is used to estimate a global average equivalent emitting temperature.
If the absorbed solar flux does not vary much over the spherical shape of a planet without an atmosphere, then using a global-average solar flux will give a pretty good estimate of the global average surface temperature.
But the absorbed solar flux actually varies a lot over a spherical planet.
So, just how large of an error is introduced by the use of a global average flux to calculate an average temperature? (My recent discussions with David South, an Auburn forestry professor, led me to reexamine this issue.)
In the case of the Moon, the error is very large. As has been pointed out elsewhere (e.g. by Willis Eschenbach here, and Nikolov & Zeller here), extreme day-night temperature swings on the Moon can cause a single-solar flux estimate of surface temperature to be biased very high, due to the nonlinearity of the S-B equation. The error can be many tens of degrees C.
Clearly, the 33 deg. C estimate for the Earth’s atmospheric greenhouse effect depends upon how accurate our estimate is of the average surface temperature of the Earth without an atmosphere. (I won’t go into the reasons why we really don’t know what the Earth would look like without an atmosphere, which affects it’s albedo and thus how much sunlight it would absorb, which in turn will impact the temperature calculation).
Since the non-linearity induced error depends upon just how hot surface temperature gets during the daytime, you need to do the calculations using a diurnal cycle, including how deep the solar heating (and nighttime cooling) penetrates into the surface. Also, obviously, the calculations need to be done on a sphere.
So, I put together this model spreadsheet that allows you to change planets (through the assumed solar irradiance), the assumed solar albedo of the atmosphere-free planet, surface longwave emissivity, and how deep of a water/soil layer is assumed to change in its temperature in response to imbalances between absorbed sunlight and thermally-emitted longwave radiation.
The time-dependent calculations are done in 96 increments of a day, which is 15 minutes for the Earth, at latitudes of 5, 15, 25, 35, 45, 55, 65, 75, and 85 degrees separately at assumed equinox conditions. Cosine latitude weighting then gives a pretty good estimate of the area averaged temperature over the sphere. It can take up to a couple weeks for the polar regions to finally equilibrate when the model is initialized at absolute zero temperature. The plots that follow are after 40 day-night cycles of the model run.
When I run the model for the Moon, which has a 29.5 Earth-day diurnal cycle, I found that I needed a soil layer of about 0.05 meters depth (about 2 inches) to match actual temperature measurements on the Moon (see Willis’s post here for some actual lunar temperature measurements). This is the thickness of soil assumed to be uniform in temperature that responds to solar heating and IR cooling. Of course, in reality the very top of the soil surface will get the hottest/coldest, with the temperature swings dampening strongly with depth; the model just uses a thin, uniform-temperature layer that approximates the average behavior of the real, thicker layer.
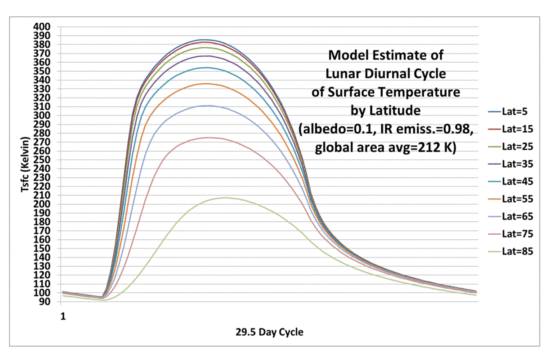
Fig. 2. Diurnal cycle in lunar surface temperatures at different latitudes calculated from a simple time-dependent model during equinox conditions.
Significantly, the resulting global area average lunar temperature of 212 K is 61 K colder than the 273 K one gets by just putting the global average absorbed solar flux through the S-B equation to get a single temperature. As discussed by Willis, this shows the large bias that can result from S-B equation calculations when one doesn’t bother to average over a wide range of temperatures.
So, How Large is the S-B Bias in Earth Temperature Calculations?
Just how big is this warm bias effect when computing what the Earth’s global average surface temperature would be in the absence of an atmosphere?
If I repeat the model calculations in Fig. 2 and only change the length of the diurnal cycle, from 29.5 Earth days (for the Moon) to 1 day, we get (obviously) a greatly reduced diurnal range in temperature (22 deg. C diurnal range, global average, versus 209 deg. C diurnal range for the Moon), and a global average surface temperature of 267 K. This is only 6 deg. below the 273 K value using a single solar flux in the S-B equation:
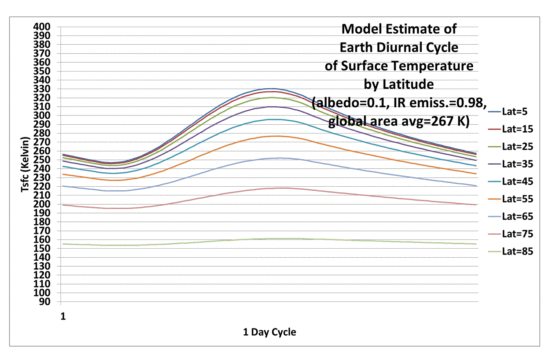
Fig. 3. As in Fig. 2, but with a 24 hr (Earth) diurnal cycle rather than 29.5 days (lunar diurnal cycle).
If I use the more traditionally-used Earth albedo value of 0.3, I get a global average surface temperature of 251 K, which is only 5 deg. C below the single solar flux calculation of 256 K. Thus, the error caused by using a single global average solar flux to estimate a global average terrestrial temperature in the S-B equation is much less for the Earth than it is for the Moon.
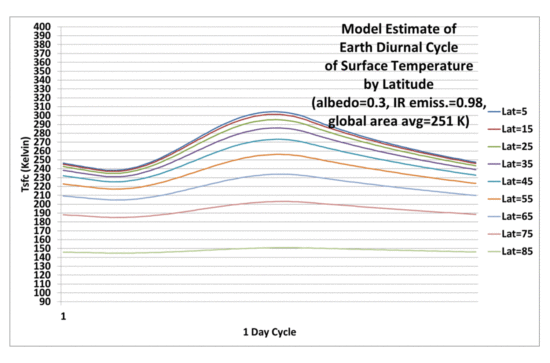
Fig. 4. As in Fig. 3, but using a solar albedo of 0.3 rather than 0.1.
Conclusion
Using the S-B equation with a global average absorbed solar flux to compute the global average emitting temperature of the Moon leads to a very large warm bias, as reported by others.
But that lunar bias (about 60 deg. C) is mostly due to the very long period of daylight on the moon, which is 29.5 times longer than on Earth. When the Earth’s diurnal cycle length is used, the warm bias is only about 5 deg. C.
One might then wonder if this means that the 33 deg. C greenhouse effect on Earth should really be 38 deg. C?
Maybe…but I would say that the 33 deg. C number is suspect anyway. First, because it depends upon an albedo of 0.3, which is probably too high. If I use a lunar albedo for the Earth, then the GHE becomes only 21 deg. C with the new calculations. One might wonder if the no-atmosphere Earth would be ice covered, with a very high albedo and very low surface temperatures, but the existence of water would lead to evaporation/sublimation, and a water vapor atmosphere. So an ice Earth is, I believe, incompatible with the assumption of no atmosphere. But I’m open to different arguments on this point.
Secondly, the 33 deg. C number isnt really the greenhouse effect, anyway. It’s more of a total “atmosphere effect”, the final result after atmospheric convection has cooled the surface substantially below the very high temperatures the greenhouse effect would cause in the case of pure radiative equilibrium (see Manabe and Strickler, 1964).
So, you can get a wide variety of numbers for the estimated surface warming effect of the atmosphere (combined effect of greenhouse warming and convective cooling). They depend on what assumptions you make in your calculations related to what an atmosphere-free Earth would look like, which are at the very least uncertain, and at most, physically impossible.
The bottom line, though, is that neglect of the nonlinearity of the S-B equation leads to about a 5 deg. C overestimate of the average surface temperature of the Earth in the absence of an atmosphere.
NOTE: Most of the comments on this post will likely be off-topic, centering around the extreme minority view of a few people that there is no atmospheric “greenhouse effect” involving the atmosphere emitting infrared radiation toward the surface.